Fuel Cell
Fuel cells are known as devices that can obtain CO2 free electricity directly from hydrogen and oxygen through chemical reactions. In our laboratory, we are working on the application of fuel cells as a new chemical reactor and the research and development of novel fuel cells by taking advantage of their unique features.
Application as a new chemical reactor
We are trying to simultaneously carry out two processes in a fuel cell: dehydrogenation from organic hydrides, which is an endothermic reaction, and power generation, which is an exothermic reaction. By studying the operating conditions (cell temperature, etc.) of the solid oxide fuel cell in detail, we have succeeded in recovering toluene from methylcyclohexane, one of the organic hydrides, while generating electricity.
This achievement shows the possibility of power generation using less energy than the dehydrogenation reaction, without using dehydrogenation facilities, which were conventionally required (Fig. 1). The recovered toluene production rate was 94%. Additionally, it has been shown that by changing the conditions, oxygen groups can be introduced into the aromatic backbone using a fuel cell.
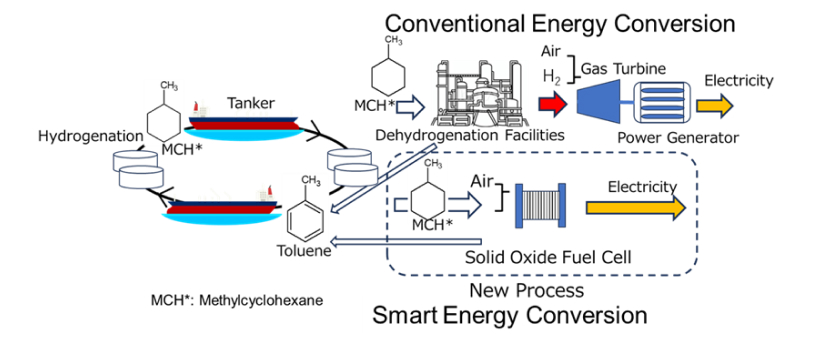
Fig. 1 New dehydrogenation and power generation reaction using fuel cells.
Development of proton-conducting ceramic fuel cells
Solid oxide fuel cells with high operating temperatures can achieve high power generation efficiency due to their high reaction activity. However, the formation of water at the fuel electrode dilutes the fuel, resulting in Nernst loss. To prevent this, proton-conducting ceramic fuel cells are attracting attention. In our laboratory, we are also investigating the effect of the intermediate layer, focusing on Ba-based electrolytes, with the aim of applying them as chemical reactors.
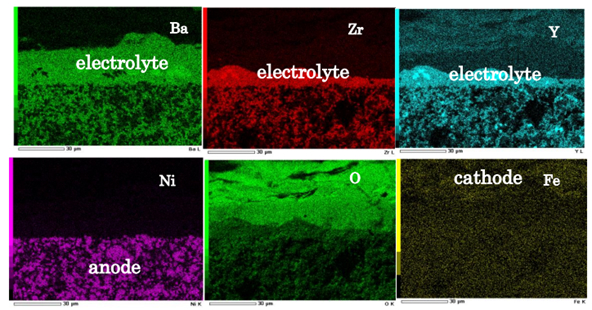
Fig. 2 Cross sectional chemical composition of proton-conducting SOFC by EDS.1)